RNA processing, Nervous system, Gene regulation, Development, RNA-binding proteins, Genetics
RNA Biology and Neurogenetics
Regulation of neuronal RNA identity and its impact on nervous system development and function
Cells utilize various mechanisms to coordinate the temporal and spatial expression of their genes. Neurons, more than any other cell type, leverage the potential of alternative RNA processing: the generation of multiple transcript isoforms from the same gene through alternative splicing and alternative 3′ end site usage. Exclusively in neurons, hundreds of genes preferentially use more distal 3′ end sites, resulting in particularly long 3′ untranslated regions (3′ UTRs). Neurons also exhibit the highest number of tissue-enriched exons and circular RNAs (circRNAs). These unique nervous-system-specific RNA sequences, collectively known as 'neuronal RNA signatures', are conserved across all animals, including humans.
The regulation of neuronal RNA signatures, how their function differs from more broadly expressed transcripts, and their impact on neuron physiology, remain open questions. Gaining insight into the biology of neuron-specific gene expression constitutes the main focus of the Hilgers Research Group (Fig. 1). As model systems, we use Drosophila melanogaster as well as cultured neurons and organoids derived from human induced pluripotent stem cells (iPSCs). Our technologies range from functional genetics, behavior studies, and imaging, to RNA biochemistry. We have a special focus on brain genomics and transcriptomics, including single-cell and ultra-long-read RNA sequencing.
Determining neuronal RNA identity
One important outstanding question is: how are brain-specific mRNA isoforms generated, and how do they contribute to nervous system function? The investigation of RNA at the isoform-level has long been hindered by technological limitations. We optimized and applied long-read RNA sequencing in such a way that we are now able to “read” entire mRNA molecules from end to end, including the very long transcripts typical for the nervous system. Our studies have identified regulatory links between gene transcription and co-transcriptional RNA processing, and have revealed that the neuron-specific RNA-binding protein ELAV is a “master regulator” of neuronal RNA identity (Fig. 2). We now focus on the genetic and epigenetic mechanisms that link transcription initiation and RNA processing, with the aim of obtaining a global understanding of how the neuron-specific RNA landscape is generated.
Regulation of the uniquely complex transcriptome of the nervous system
Targeted transport, packing into ribonucleoprotein granules, and local translation, have emerged as key mechanisms controlling cellular function. We study how neuronal RNA signatures regulate these processes specifically in the nervous system. For example, we identified neuronal 3′ UTR regulation as an important mechanism that balances protein abundance in conditions of stress. We also showed that tissue-specific RNA-protein interactions can control the spatial expression of synaptic proteins (Fig. 3). Several of our ongoing projects address how neuron-specific RNAs modify the behavior of biomolecules (RNAs and proteins) to achieve meaningful physiological function.
Functional impact of RNA isoform diversity in the brain
The modification of the neuronal RNA landscape can impact protein function in a context-, gene-, or cell-type-specific manner. Our work has uncovered a global role for neuronal RNAs in the maintenance of protein functionality during development and in conditions of physiological duress: their depletion causes neurodevelopmental defects, adult brain dysfunction, and phenotypes of neurodegeneration. We generated Drosophila and human disease models, in which we can acutely alter levels of neuronal RNAs in the brain. These and other genetic models that we are establishing in the lab will empower the molecular study of brain processes in normal and altered states of neuronal RNA expression.
Connection to Clinical Practice
RNA signatures are key players in normal functioning of neuronal cells. Faulty RNA processing is emerging as a hallmark of neurodevelopmental disorders, and neurodegeneration phenotypes. The extreme diversity and complexity of the RNA landscape in neurons is not only a strength, but also a vulnerability that may contribute to the penetrance of pathologies of the nervous system.
Unravelling the molecular processes underlying neuronal RNA regulation will lead to the identification of pathogenic mechanisms that can be targeted and possibly reversed. For example, 3′ UTR genetic variants contribute to a substantial number of phenotypic traits and disease heritability, making neuron-specific RNA biogenesis an attractive target for therapeutic intervention.
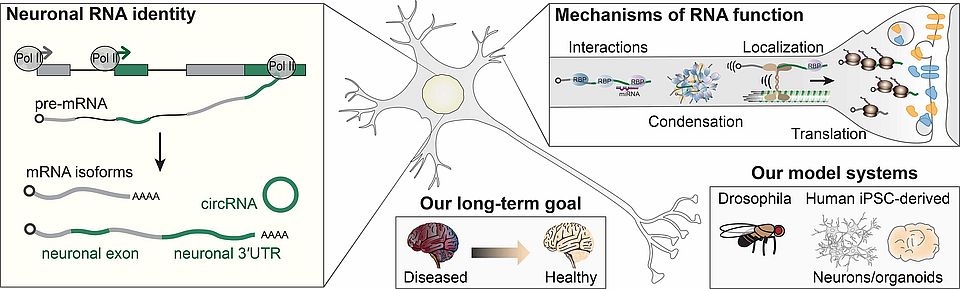
Fig. 1: Overview of our research. We investigate the mechanisms that lead to the emergence of the neuron-specific RNA landscape, the post-transcriptional regulation of neuronal RNA signatures, and their role in neuronal development and function. Left: In the nucleus, ELAV binds to the nascent RNA and co-transcriptionally inhibits splicing and 3′ end processing to produce neuronal transcript signatures. Right: in the cytoplasm, RNA-binding proteins specifically bind neuronal RNA sequences to direct isoform-dependent interactions, biomolecular condensation, localization, and translation. Disruption of these neuron-specific RNA processes can lead to neurological disease, which we study using animal (the fruit fly Drosophila melanogaster) and in vitro (human iPSC-derived neuronal cultures) models of the brain. Our long-term goal is to target neuronal RNA mechanisms and counteract phenotypes of disease.
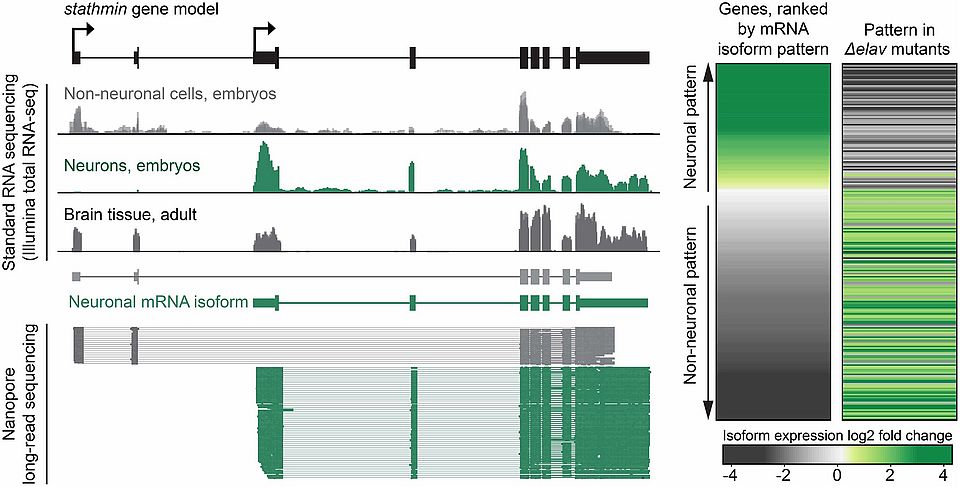
Fig. 2: Defining neuronal RNA identity using transcriptomics. Left, neuronal gene expression patterns revealed by short-read RNA-seq (top gene tracks) and long-read RNA sequencing for the example gene stathmin in Drosophila. In neurons, mRNA signal strongly differs from that in other, “non-neuronal” cells. Long-read-sequencing allows to distinguish, in the same tissue, neuron-specific (green) and more broadly expressed (grey) isoforms from end to end. Right, ranking of gene isoforms (about 1000 genes, each line is one gene) according to the specificity of their neuronal expression pattern (“neuronality”. In neurons lacking the protein ELAV (Δelav), transcripts lose their typical neuronal identity.
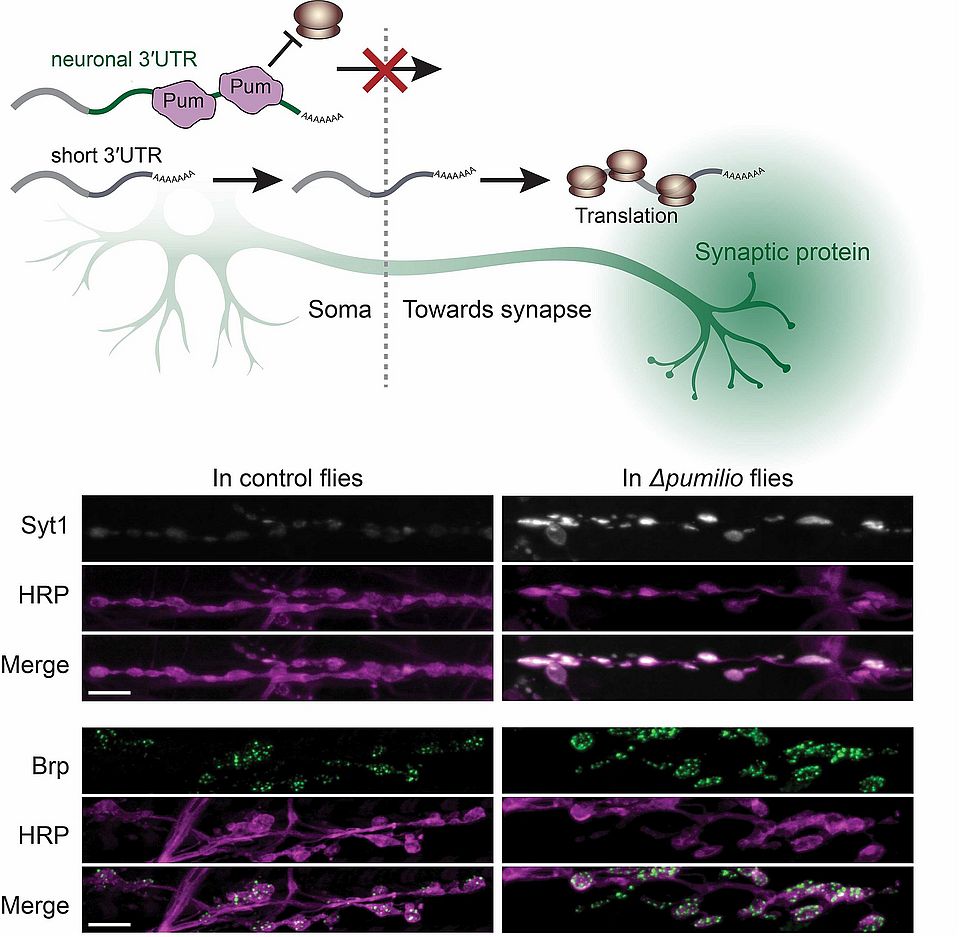
Fig. 3: Regulation of synaptic protein expression through neuron-specific RNA sequences. Top: Mechanism of differential regulation of mRNAs carrying neuron-specific 3′ UTRs. In neuronal cell bodies, the translational repressor Pumilio binds to mRNAs encoding synaptic proteins, with a preference for binding long 3′ UTR isoforms (carrying neuron-specific 3′ UTRs). This leads to the enriched localization of translationally competent short isoforms in synaptic compartments, and synaptic expression of the encoded protein. Bottom: Confocal imaging showing neuromuscular junctions of control flies and mutants lacking the protein Pumilio (Δpumilio). Loss of Pumilio causes overexpression of the synaptic proteins Synaptotagmin 1 (Syt1) and Bruchpilot (Brp) at synaptic boutons. HRP marks presynaptic membranes. Scale bars: 10 μm.