Multiple Sclerosis . Autoreactive B-Cells . Treatment Response . Prognostic Biomarkers . Pathophysiology of Progressive MS . Precision Medicine
Clinical Neuroimmunology
Molecular and immunological analysis of Multiple Sclerosis and related disorders
Multiple sclerosis (MS) is an inflammatory and neurodegenerative disease of the central nervous system (CNS). It is the number one cause of neurological disability in young adulthood, eventually leading to various degrees of long-term deterioration ("progression") of cognitive and somatic functions despite the almost complete suppression of acute exacerbations ("relapses") by modern therapy; the molecular mechanisms leading to progression of MS are poorly understood. We pursue two main research lines:
1) to elucidate the role of B-cells and antibodies in this process,
2) to explore and develop biomarkers that capture and prognosticate disease course and reflect therapy response for individual therapeutic decision making, i.e. pursue the translation from 'precision medicine' to 'personalized medicine'). In recent years diseases (neuromyelitis spectrum disorders (NMOSD) and myelin oligodendrocyte glycoprotein asociated disease (MOGAD)) that were until recently subsumed under the diagnosis of "MS" have been defined as separate entities, based on the recognition distinct pathomechanisms. The validation of biomarkers for differential diagnosis of MS from other diseases and for drug response is a further pillar of our research.
B cells and their targets in MS (Derfuss group)
B-cells have a major role in the pathogenesis of MS. Depletion of B-cells leads to a remarkable amelioration of the disease. The mechanisms by which B-cells impact MS are however incompletely understood. Our research focuses on the identification of novel B-cell autoantigens and the characterization of the interaction of auto-aggressive B-cells with the CNS. We could show that antibodies against native myelin oligodendrocyte glycoprotein (MOG) identify a subset of patients with neuromyelitis optica and are also found in SLE patients with CNS involvement (Pröbstel et al., 2015; Pröbstel et al., 2019). Using transgenic animal models, we were able to prove a new concept how infection could lead to the development of autoantibodies like those against MOG. We identified the co-capture of membrane antigens by the B cell receptor as a key step in initiating an autoimmune response in the context of an infection (Sanderson et al., 2017). This work is now continued by analyzing the capacity of B cell to migrate to peripheral tissues including the CNS and harvest their cognate and non-cognate antigens from the tissue. The phenomenon of membrane capture can also be used to purify anti-viral B cells from blood (Zimmermann et al., 2019; Callegari et al., 2022), to clone autoantibodies against the acetylcholine receptor in myasthenia gravis patients (Rose et al., 2022) and to identify novel B cell autoantigens in MS.
Using samples and clinical data from the Swiss MS Cohort study (smsc.ch) we could identify immunological predictors for treatment response and risk of immunological side effects in MS patients treated with dimethylfumarate (Diebold et al., PNAS 2022; Diebold et al., Ann Neurol 2022)
Defining biomarkers for disease progression and therapy response (Kuhle group)
Neurofilament light chain (NfL) is a real-time biofluid marker specific for neuronal damage and has been established by us as a blood-based precision medicine tool to measure disease activity and drug response (Fig. 2A), and to predict the long-term outcome of disability. The establishment of normative data for adults (https://shiny.dkfbasel.ch/baselnflreference/) and children’s (https://shiny.dkfbasel.ch/baselnflreference-for-kids) individual NfL data can now be used for individual therapeutic decision making, as well as a drug-response endpoint in clinical trials.
Glial fibrillary acidic protein (GFAP) is an astrocyte-specific biomarker whose increase in blood reflects the "sclerosis" (astrocyte proliferation) part of MS pathogenesis. We have found that the combination of NfL and GFAP measurement has now become the promising prognosticator of disability worsening (Fig. 2B), and of drug response in MS on the group level. We aim now for extension and validation of our results in large and longitudinal cohorts of patients, and the establishment of normative values for GFAP, alike for NfL, to allow the individual use in routine clinical practice.
We have recently established granulocyte activation products as biomarkers that allow the distinction of NMOSD from MS with better sensitivity than the current diagnostic gold-standard. As the diagnosis of NMOSD requires immediate therapy, different from MS, we aim at their development towards a same-day diagnostic tool to meet this need.
Further, we investigate other biofluid markers by several proteomic platforms to contextualise established biomarker signals with regard to state and stage of MS, and for differential diagnosis vis-à-vis other inflammatory neurological diseases. These projects are based on patient information and biological samples from the Swiss MS Cohort Study (smsc.ch), a consortium of Swiss academic MS centres founded in 2012, and international collaborations in Europe the Nothern Americas and Asia.
Connection to Clinical Practice
Our two research groups are closely connected to the MS Centre and Outpatient Clinic of the Department of Neurology, University Hospital Basel that provides care for more than 1350 MS patients per year. The MS Centre plays a key role in conceptualizing and conducting international therapeutic trials to bring novel therapies to persons with MS. These trials provide unique possibilities for a translational medicine approach by connecting basic research and clinical studies for a better understanding of disease, and specifically for progressive MS.
The better understanding and treatment of MS needs prospective, standardized, and high-quality MRI and clinical data from large patient cohorts to allow for development and validation of biomarkers on the individual patient level, and hence application in clinical practice as Precision Medicine tools. The Swiss MS Cohort Study (SMSC; smsc.ch), initiated in 2012 and coordinated by the MS Centre Basel since then, is the mainstay of research efforts in Clinical Neuroimmunology; it provides an internationally unique long-term follow-up of over 1400 Swiss MS patients with clinical and MRI data, and blood and cerebrospinal fluid samples for research. The SMSC provides access to a population of MS-patients in all stages and states of disease, treated with all state-of-the-art therapies. The close collaboration with the Translational Imaging in Neurology (ThINk) group at the Department of Biomedical Engineering enables characterization of patients with cutting edge neuroimaging techniques.
Figure 1
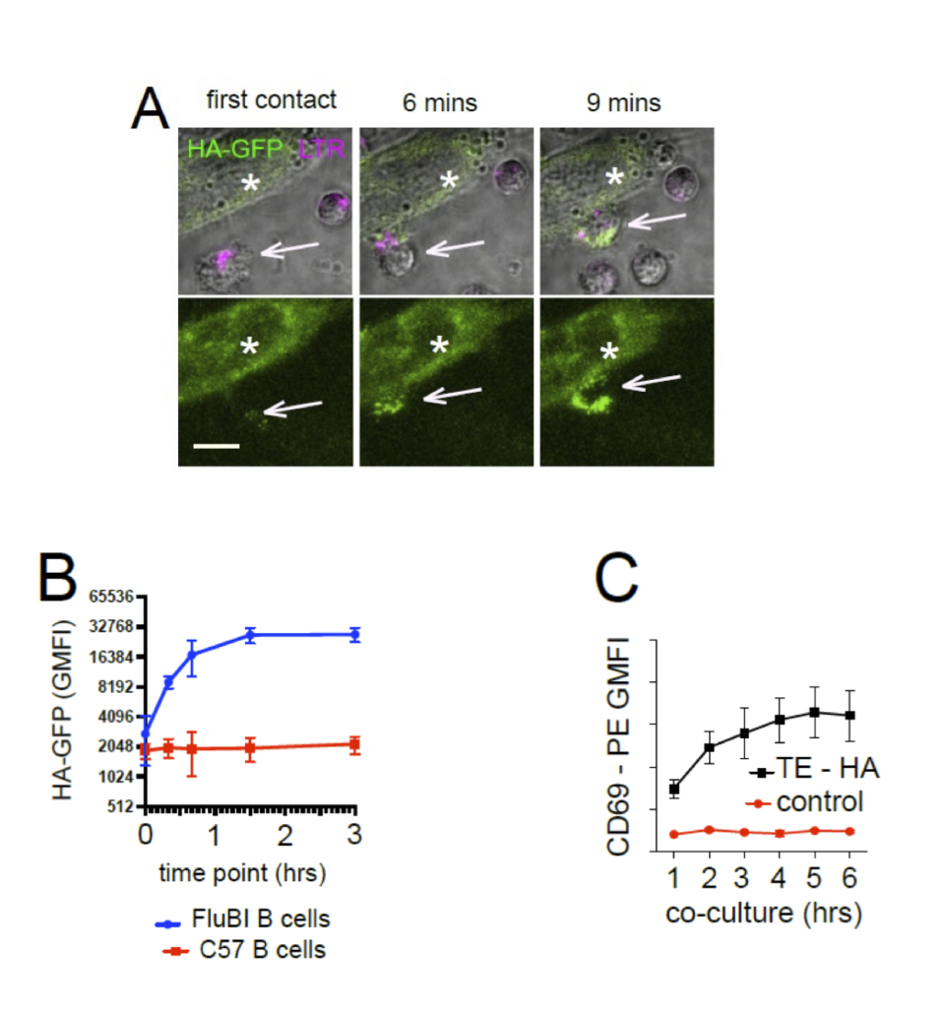
Antigen Extraction from Membrane by Cognate B cells (A) Live Cell Imaging of membrane antigen capture. Hemagglutinin-specific FluBI mouse B cells (white arrows, labeled magenta LTR) are added to cells (white asterisks) expressing a fusion protein of hemagglutinin with a cytoplasmic GFP (HA-GFP, green). Images show the initial contact between a B cell and an antigenic target cell, then the same location at 6 and 9 minutes later. The lower panels show only the GFP channel to show capture of antigen (scale bar = 10 um). (B) Extraction of membrane expressed HA-GFP by antigen-specific or antigen-irrelevant B cells. HA-specific FluBI B cells were labeled with Cell Trace Violet (CTV), mixed with unlabeled, antigen irrelevant mouse B cells at a ratio of 1:10, and added to an adherent layer of cells stably transfected with membrane-expressed HA-GFP. At the indicated time points, the B cells were retrieved and interrogated by flow cytometry. The FluBI cells were separated from unspecific B cells by CTV label, and GFP levels were compared between the two cell types. Points and bars show mean and standard deviation of the geometric mean GFP fluorescence. (C) Time-course of CD69 upregulation. HA-specific FluBI mouse B cells were exposed to HA-expressing TE HA, or HA-non-expressing control cells for the indicated times, and then retrieved, immunolabeled for B220 and CD69 and measured by flow cytometry. Vertical axis shows the geometric mean immunofluorescence intensity and SEM of the anti-CD69.
Fig 2A Temporal evolution of sNfL Z scores under three levels of efficacy of MS therapy (Benkert et al., Lancet Neurology, 2022)
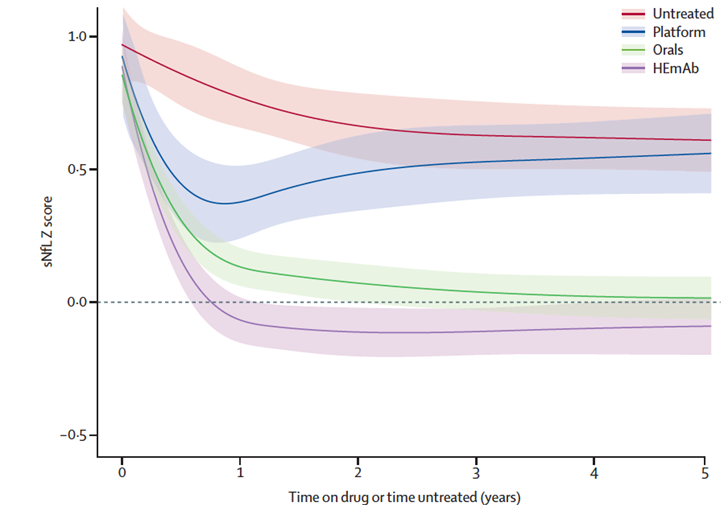
Four treatment categories were included in a mixed-effects model, thereby using spline terms to model the non-linear temporal association and an interaction term between disease-modifying therapy category and treatment duration. Shaded areas indicate 95% CI. HEmAb=high efficacy monoclonal antibody therapies.
Fig 2B Combined Biomarker Data of NfL and GFAP to Predict Time to Confirmed Disease Worsening (CDW) (Meier et al., Jama Neurology, 2023)
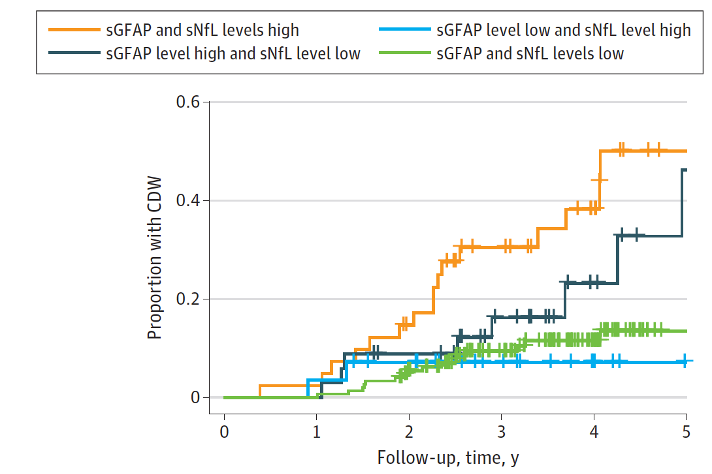
Optimized cutoffs of serum GFAP and NfL z scores from receiver operating characteristic curve analysis, based on the Youden index, were used to dichotomize patient groups. High sGFAP/high sNfL levels were associated with a 4-fold (hazard ratio[HR], 4.09; 95%CI, 2.04-8.18; P< .001) increased risk of CDW, compared with low sGFAP/low sNfL levels. The combination of high sGFAP/low sNfL levels showed a slightly reduced risk (HR, 2.32; 95%CI, 0.99-5.42; P=0.05).