Myelin Biology . Axon-Glia Interaction . Membrane Domains and Trafficking Multiple Sclerosis . Peripheral Neuropathy Neuroprotection
Neurobiology
Molecular Mechanisms of Myelin Formation and Maintenance in Health and Disease
An Animal Model for Chronic Meningeal Inflammation and Inflammatory Demyelination of the Cerebral Cortex
Modeling chronic cortical demyelination allows the study of long-lasting pathological changes observed in multiple sclerosis such as failure of remyelination, chronically disturbed functions of oligodendrocytes, neurons and astrocytes, brain atrophy and cognitive impairments. We aimed at generating an animal model for studying the consequences of chronic cortical demyelination and meningeal inflammation (Fig. 1). To induce long-lasting cortical demyelination and chronic meningeal inflammation, we immunized female Lewis rats against myelin oligodendrocyte glycoprotein (MOG) and injected lentiviruses for continuing overexpression of the cytokines NFα and IFNγ in the cortical brain parenchyma. Immunization with MOG and overexpression of NFα and IFNγ led to widespread subpial de-myelination and meningeal inflammation that were stable for at least 10 weeks. We demonstrate here that immunization with MOG is necessary for acute as well as chronic cortical demyelination (Fig. 2). In addition, long-lasting over-expression of NFα and IFNγ in the brain parenchyma is sufficient to induce chronic meningeal inflammation. Our model simulates key features of chronic cortical demyelination and inflammation, reminiscent of human multiple sclerosis pathology. This will allow molecular, cellular and functional investigations for a better understanding of the adaptation mechanisms of the cerebral cortex in multiple sclerosis.
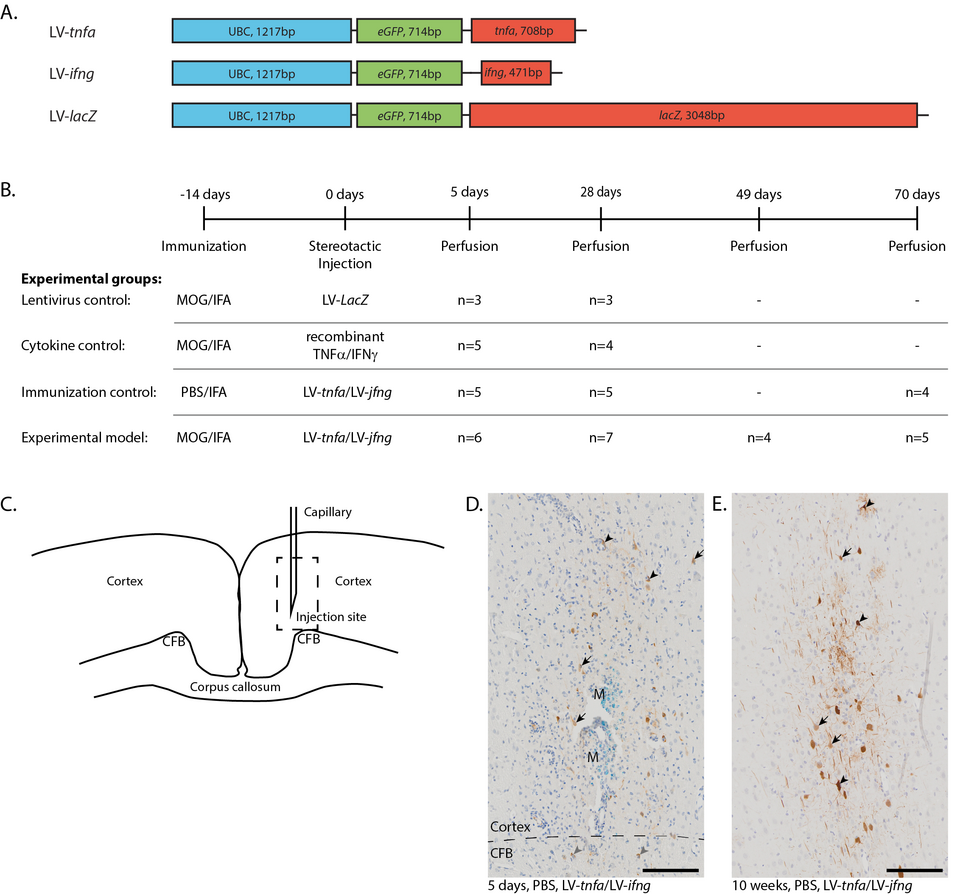
Fig. 1: NFα and IFNγ Is Required for Meningeal Inflammation. Five days after stereotactic injection, a widespread meningeal infiltrate was identified in all animals chronically overexpressing TNFα and IFNγ, irrespective of MOG immunization (Figure A1A–D, arrows). The mean maximum thickness of meninges was 99.1 μm in animals chronically overexpressing TNFα and IFNγ compared to 16.8 μm in animals Figure 1. Lentiviral constructs and experimental design. (A) Schematic drawing of the lentiviral constructs depicting the human ubiquitin C promoter (UBC) and the sequences for enhanced green fluorescent protein (eGFP), tumor necrosis factor alpha (tnfa), interferon gamma (ifng), and beta galactosidase (LacZ). (B) Experimental timeline and table depicting the four experimental groups with the respective number of animals analyzed per study group and time point. Animals of the first two time points are pooled from two independent experiments. (C) Sketch of the injection site. Dashed box: approximate location of the images shown in (D,E). (D) Anti-GFP staining five days after the LV-tnfa/LV-ifng injection reveals cells of neuronal (arrows) and glial morphology in the cortex (black arrowheads) and in the cingulate fiber bundle (CFB, grey arrowheads). The tracer monastral blue (M) is detected along the injection trajectory. Dashed box: area of inset. Inset is at 160% magnification. (E) Anti-GFP staining 10 weeks after the LV-tnfa/LV-ifng injection shows cells of neuronal (arrows) and glial (arrowheads) morphology. Dashed box: area of inset. Inset is at 160% magnification. Scale bars: 100 m. Abbreviations: MOG: recombinant myelin-oligodendrocyte glycoprotein, PBS: phosphate-buffered saline, IFA: incomplete Freund’s adjuvant, CFB: cingulate fiber bundle, M: monastral blue.
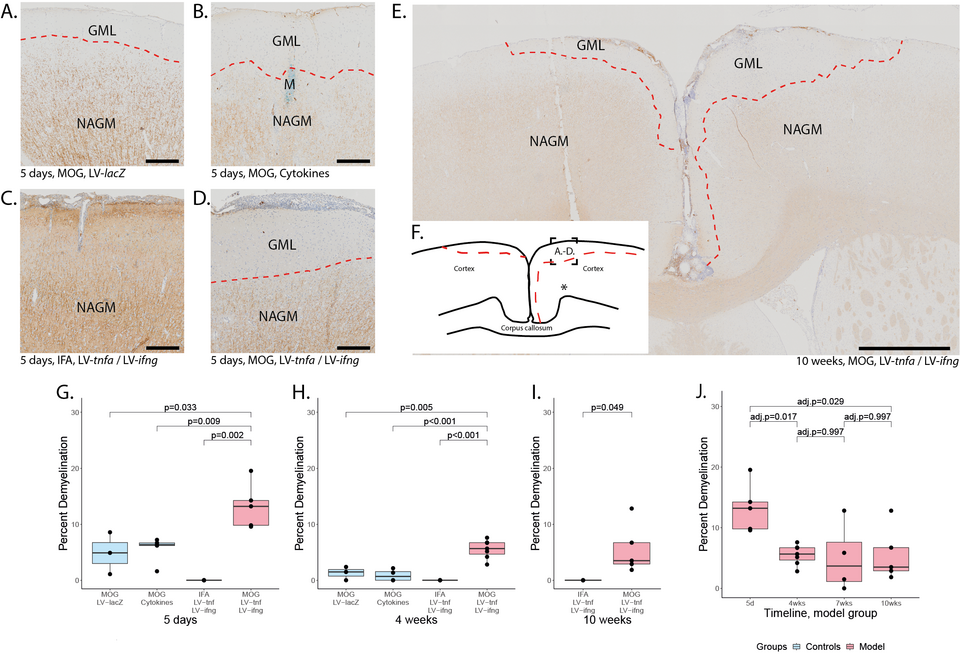
Fig. 2. Subpial demyelination. Representative images of anti-MBP stainings demonstrating the subpial demyelination five days after the intracerebral injection of (A) LV-LacZ, (B) recombinant protein TNF and IFN, (C) LV-tnfa/LV-ifng in non-immunized animals and (D) LV-tnfa/LV-ifng in MOG-immunized animals. (E) Overview image representing the subpial demyelination observed 10 weeks after the intracerebral injection. (F) Representative illustration of observed cortical demyelination pattern (dotted lines) 10 weeks after intracortical injection (asterisk). Dotted square shows the localization of the images in (A–D). (G–I) Quantification of demyelinated area: Boxplots show the percent of demyelinated area across both hemispheres for all study groups, five days, four weeks, or ten weeks after lentiviral injection. A two-sided Welch t-test was performed to compare between groups. (J) Timeline of data presented in (G–I) of the MOG-immunized, LV-tnfa/LV-ifng-injected animals. An ANOVA and Tukey post hoc test were performed to compare the different time points. The data of the boxplots are described in detail in Table A5. Scale bars (A–D): 100 m, (E): 500 m. Abbreviations: GML: grey matter lesion; NAGM: normal-appearing grey matter.
Theophylline Induces Remyelination and Functional Recovery in a Mouse Model of Peripheral Neuropathy
Charcot-Marie-Tooth disease (CMT) is a large group of inherited peripheral neuropathies that are primarily due to demyelination and/or axonal degeneration. CMT type 1A (CMT1A), which is caused by the duplication of the peripheral myelin protein 22 (PMP22) gene, is a demyelinating and the most frequent CMT subtype. Hypermyelination, de-myelination, and secondary loss of large-caliber axons are hallmarks of CMT1A, and there is currently no cure and no efficient treatment to alleviate the symptoms of the disease. We previously showed that histone deacetylases 1 and 2 (HDAC1/2) are critical for Schwann cell developmental myelination and remyelination after a sciatic nerve crush lesion. We also demonstrated that a short-term treatment with Theophylline, which is a potent activator of HDAC2, enhances remyelination and functional recovery after a sciatic nerve crush lesion in mice. In the present study, we tested whether Theophylline treatment could also lead to (re)myelination in a PMP22-overexpressing mouse line (C22) modeling CMT1A. Indeed, we show here that a short-term treatment with Theophylline in C22 mice increases the percentage of myelinated large-caliber axons (Fig.3) and the expression of the major peripheral myelin protein P0 and induces functional recovery. This pilot study suggests that Theophylline treatment could be beneficial to promote myelination and thereby prevent axonal degeneration and enhance functional recovery in CMT1A patients.
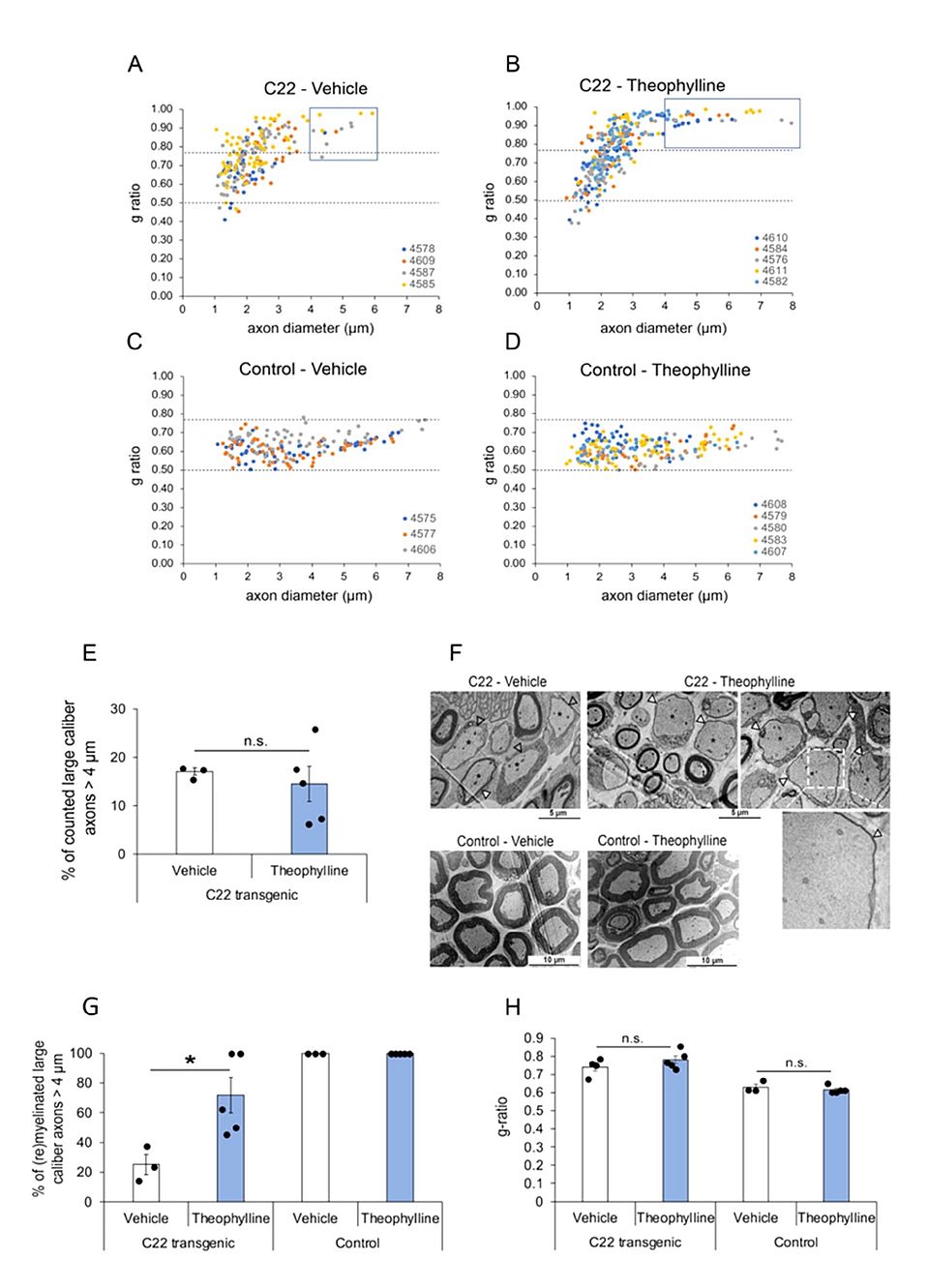
Fig. 3: Theophylline induces (re)myelination of large-caliber axons. G-ratio distribution in sciatic nerves of C22 transgenic and control mice treated with Theophylline or vehicle. G-ratio/axon diameter scatter diagrams of sciatic nerve axons from C22 transgenic (A,B) and control (C,D) mice treated with Theophylline or vehicle as shown in Figure 1A. The data points of one animal (each identified by a unique number) are in the same color. Values above 0.77 indicate hypomyelinated axons, while values below 0.50 indicate hypermyelinated axons (delineated by dashed lines). For C22 transgenic mice treated with vehicle (A), the g-ratio of 203 axons is plotted (n = 4 mice, 25–80 axons per mouse). For C22 transgenic mice treated with Theophylline (B), the g-ratio of 308 axons is plotted (n = 5 mice, 35–79 axons per mouse). For control mice treated with vehicle (C), the g-ratio of 178 axons is plotted (n = 3 mice, 55–62 axons per mouse). For control mice treated with Theophylline (D), the g-ratio of 214 axons is plotted (n = 5 mice, 31–65 axons per mouse). The blue boxes in (A,B) indicate large-caliber axons > 4 m. (E) Graph representing the percentage of counted large-caliber axons (>4 m diameter) in sciatic nerves of C22 mice treated with vehicle or Theo-phylline. (F) Representative electron micrographs of sciatic nerves of C22 or control mice treated with vehicle or Theophylline. The dashed white box on the top right image highlights the region corresponding to the magnified image underneath showing a thin myelin sheath (filled arrowhead) around this largecaliber axon. (G) Graph representing the percentage of (re)myelinated large caliber axons (>4 m) in sciatic nerves of C22 or control mice treated with vehicle or Theophylline. Unpaired two-tailed Student’s t-test, n = 3 for vehicle-treated C22 or control mice and 5 for Theophylline-treated C22 or control mice. (H) Graph representing the average g-ratio of all counted axons in sciatic nerves of C22 or control mice treated with vehicle or Theophylline. * p value < 0.05, n.s.: statistically non-significant. (E,H) Unpaired one-tailed Student’s t-test, n = 3 for vehicle-treated C22 or control mice and 5 for Theophylline-treated C22 or control mice. Black dots show individual data points.
New publications
Enz, L.S.;Winkler, A.; Wrzos, C.; Dasen, B.; Nessler, S.; Stadelmann, C.; Schaeren-Wiemers, N. An Animal Model for Chronic Meningeal Inflammation and Inflammatory Demyelination of the Cerebral Cortex. Int J Mol Sci. 2023 Sep 9;24(18):13893. doi: 10.3390/ijms241813893. PMID: 37762198
Mert Duman, Stephanie Jaggi, Lukas Simon Enz , Claire Jacob and Nicole Schaeren-Wiemers. Theophylline Induces Remyelination and Functional Recovery in a Mouse Model of Peripheral Neuropathy Biomedicines. 2022 Jun 15;10(6):1418. doi: 10.3390/biomedicines10061418. PMID: 35740439
Lukas Simon Enz, Stephanie Jaggi, Paula Huemer and Nicole Schaeren-Wiemers. The Role of Whole Genome Gene Expression Studies in Deciphering Cortical Grey Matter Pathology in Multiple Sclerosis. 2021. J Clin Exp Neuroimmunol. 6: 125. Doi: 10.4172/jceni.1000125
Enz LS, Zeis T, Schmid D, Geier F, van der Meer F, Steiner G, Certa U, Binder TMC, Stadelmann C, Martin R, Schaeren-Wiemers N. Increased HLA-DR expression and cortical demyelination in MS links with HLA-DR15. Neurol Neuroimmunol Neuroinflamm. 2019 Dec 27;7(2). pii: e656. doi: 10.1212/NXI.0000000000000656. Print 2020 Mar. PubMed PMID: 31882398.
Enz LS, Zeis T, Hauck A, Linington C, Schaeren-Wiemers N. Combinatory Multifactor Treatment Effects on Primary Nanofiber Oligodendrocyte Cultures. Cells. 2019 Nov 12;8(11). pii: E1422. doi: 10.3390/cells8111422. PubMed PMID: 31726669; PubMed Central PMCID: PMC6912369.